CCI Light Damage Calculator 2 – Multipoint Source Data
Eric Hagan, Canadian Conservation Institute
Background
The second version of the CCI Light Damage Calculator (LDC) ‒ described in HERIe as ‘CCI Light Damage Calculator 2’ ‒ uses spectrophotometer measurements of visible-reflectance versus light dose for different types of materials in heritage collections. The illuminant used for light exposure is a warm white (3000K) LED with high colour rendering (CRI>=95), typical of modern electric gallery lighting. Source data typically consist of 20 to 30 measurements up to a final light dose of 85 to 100 mega lux-hours (Mlx·h). Reflectance spectra are converted to colour values corresponding to the CIE D65 illuminant for a 2° observer, which are then used to render colour patches and calculate CIEDE2000 colour difference (∆E00) based on user-selected light exposure conditions. The calculator presently interfaces with a single published dataset for early synthetic dyes on textiles [1,2]. As further material datasets with similar structures are developed, they will be incorporated in the tool as user-selectable material categories. A brief description of the tool is provided below, starting with a comparison to the original CCI Light Damage Calculator [3] ‒ indicated in HERIe as ‘CCI Light Damage calculator 1’.
Comparison with the original CCI Light Damage Calculator
This new version of the LDC was developed to accurately represent the colour changes of materials in heritage collections when exposed to an illuminant typical of gallery lighting. This offers more precise data for professionals focused on specific materials. Most importantly, this version addresses the main practical limitation of the original by incorporating broader statistics for material categories. When reflecting on the original LDC, Michalski [4] mentions the focus on single colourants as its main limitation when considering the practical requirements of those engaged in broad risk assessments of collections. Each version of the Light Damage Calculator has unique advantages and disadvantages; therefore, both are currently implemented in HERIe. These are briefly outlined below.
Original CCI Light Damage Calculator – single point source data
Advantages:
- Lightweight and simple to implement in software
- No maximum limit with respect to light dose since a mathematical equation is implemented for the calculations of colour values
- Use of historical (published) data for materials in heritage collection—initial colour and a lightfastness (Blue Wool) rating to define the initial rate of colourant loss.
Disadvantages:
- Colour change is assumed to follow a theoretical function that represents colour loss, and all materials fade to white. Some materials may not follow the function. They may change in hue—for example with dye mixtures where components change at different rates. Other materials may darken with light exposure—for example, wool dyed with picric acid.
Ver. 2 Light Damage Calculator – multipoint source data
Advantages:
- Accurate representation of colour change with light exposure using a test illuminant representative of modern gallery lighting
- Precise colour measurements facilitate calculations of changing lightfastness of residual colours given prior exposure (a feature under development)
- Larger datasets for various study materials can facilitate the representation of lightfastness statistics for that material category. This is particularly useful for professionals broadly assessing lighting risk to collections where the individual materials are not known.
Disadvantages:
- User-selected light dose values are limited to the range of measurements in source data (typically 85 to 100 Mlx·h)
- More difficult to implement due to the larger dataset and need for interpolation between measurements based on user-selected dose values.
Calculations
Figure 1 shows an example set of visible reflectance spectra measured from a dyed textile at several light dose values. These spectra are useful for other research applications such as colour rendering and colour perception. For a light damage calculator, our primary interest is the associated colour values. These are precalculated for each measurement increment and stored in the database with spectral data.
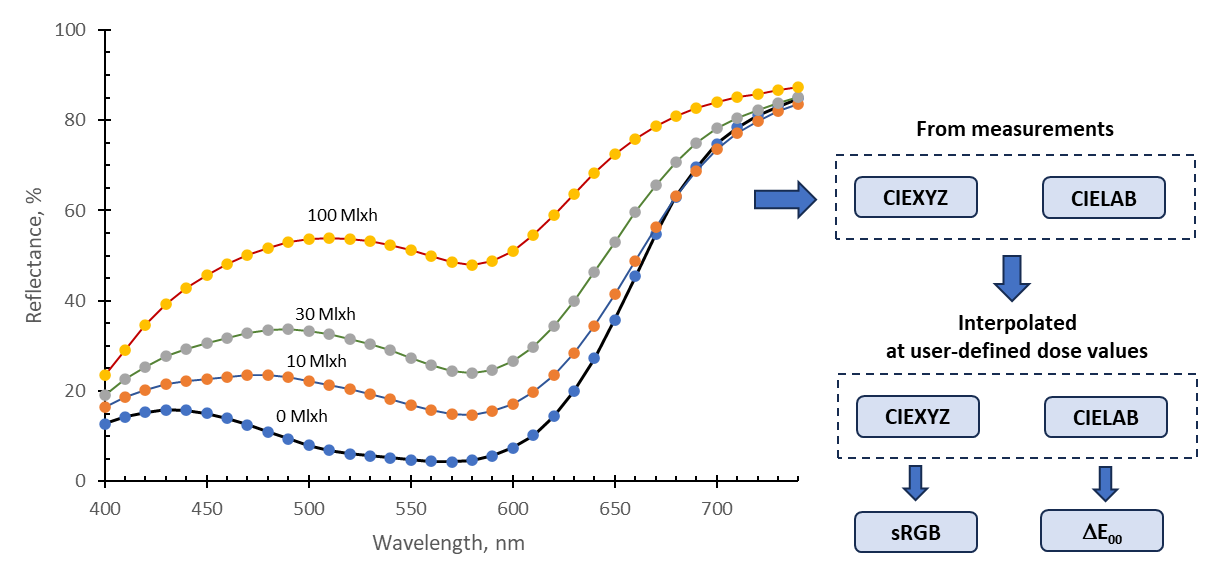
When a user enters light exposure values for prior and future dose, the calculator interpolates the respective colour values from the dataset and performs further calculations for CIEXYZsRGB (visual colour swatches) and paired CIELAB∆E00 (colour difference). Figure 2 shows the calculated CIEXYZ and CIELAB values from each vis-reflectance spectrum for a standard D65 illuminant with a 2° observer, using the full dataset for the dyed textile. Vertical lines show examples of where the values would be interpolated for a case where the user selects 10 Mlx·h for the prior exposure (defining the current colour), and a subsequent 20 Mlx·h of further exposure (defining the forecasted future colour). The CIEXYZ values are then converted to sRGB values for rendering colour swatches, and the CIELAB values are used to calculate colour difference.
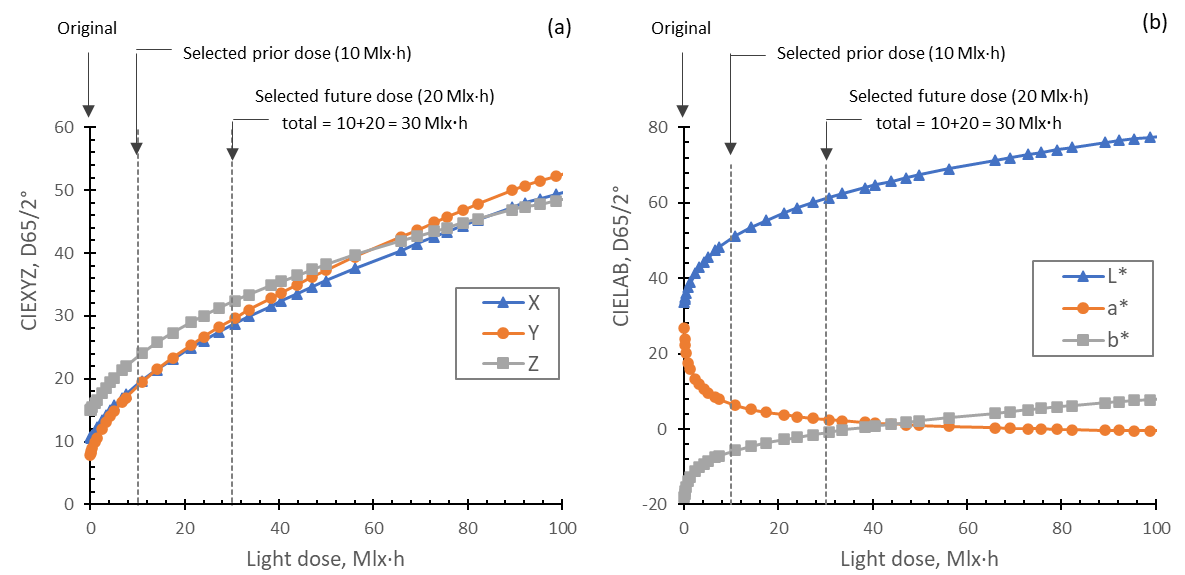
Figure 3 displays a series of colour swatches, rendered in sRGB, derived from interpolated CIEXYZ values at the respective dose values of 0, 10, and 30 Mlx·h. The last swatch estimates the residual colour after a future exposure calculated from the monitoring data uploaded by the user. It is assumed that the uploaded data represents one year and the yearly dose is calculated. Any missing values and gaps in the data are linearly interpolated. The future light dose is then calculated as the yearly dose multiplied by the number of years selected. Alternatively, the forecasted future exposure is calculated ‒ as the pre-exposure ‒ from a selected number of years, light intensity, number of exposure days per year, and number of exposure hours per day. The original (unexposed) colour is used as a point of reference for assessing colour change at a current 10 Mlx·h pre-exposure, and also at an additional 20 Mlx·h forecasted future exposure (30 Mlx·h total). Colour difference is similarly assessed between the current and future colour swatches. Numerical values for colour difference, dose and colour are shown below the swatches for reference.
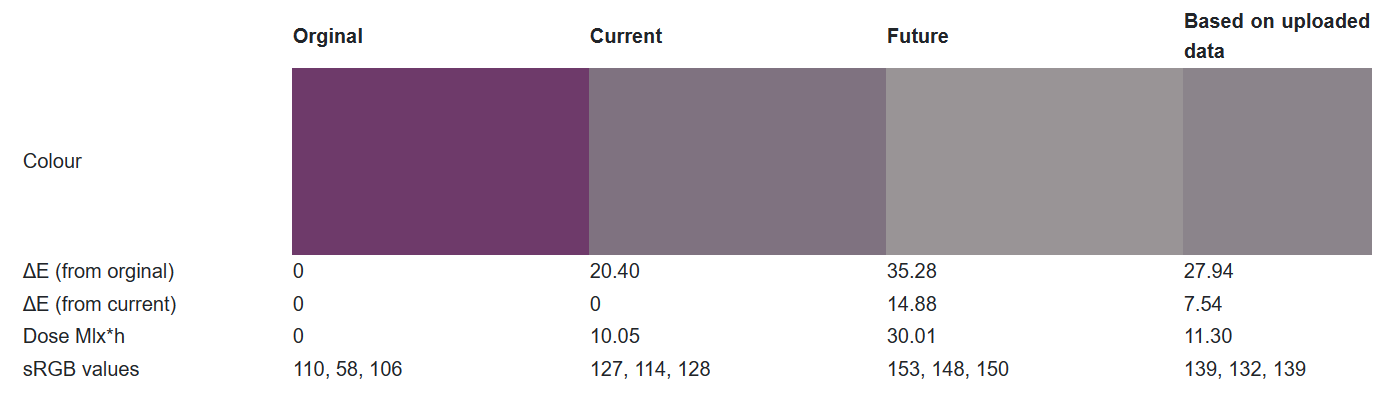
The lightfastness values of the original and residual colours in Figure 3 are not currently calculated in HERIe; however, they can be approximately assessed by moving the sliders to a dose that gives ∆E1.7. This is the dose causing a just-noticeable difference (JND), which is equivalent to an ISO Grey Scale [5] contrast of 4 (GS4).
It is helpful to consider not only the light sensitivity of the pristine material, but also that of the residual colours that remain in collections. A simple visual comparison between the original colour and the residual after 10 Mlx·h indicates a substantial amount of colour change. At this state, we are well past the point of preserving the original colour of the dyed textile; however, preserving the remaining colour is important for maintaining contrast, and ensuring the visibility of images and patterns on an object. For instance, consider the upholstered furniture in Figure 4 located in a historic house. The textile is highly faded due to prior exposure, but the details of the decorative work remain evident because of the surviving colours. When assessing the risk of colour change from the current state, attributing the same sensitivity class as a similar pristine object would be overly conservative [6]. Instead of being in the high sensitivity range of Blue Wool (BW) 1-3, the diminished rate of colour change may place it in the BW4 or higher category (medium sensitivity).
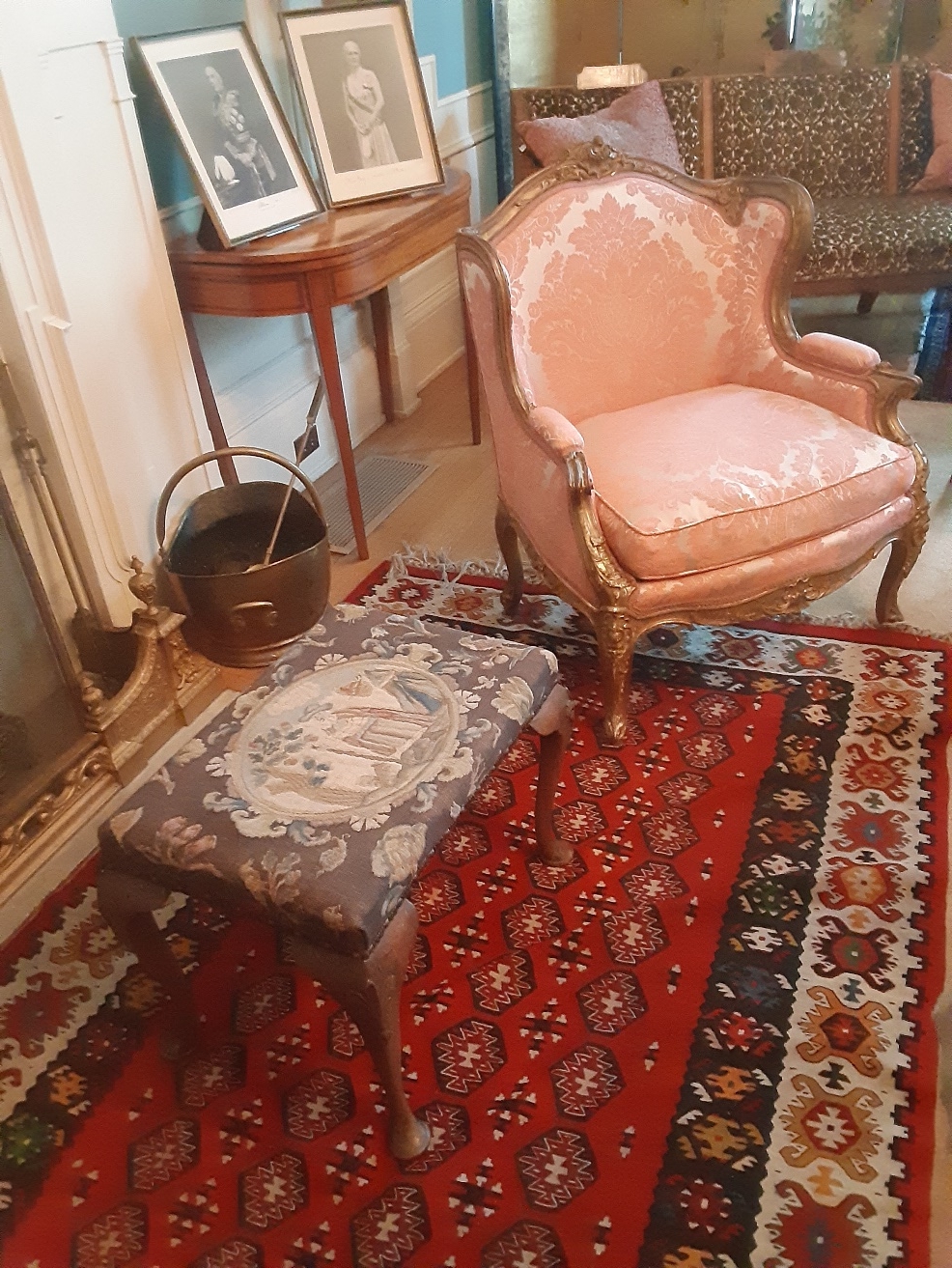
To further illustrate this concept, Figure 5a presents trends of colour change, ∆E00, with light exposure, starting from two reference points: 1. the original, unexposed material; and 2. the residual colour following 10 Mlx·h of prior exposure. These points correspond to the user-selected dose scenario depicted in Figure 2, illustrated by the colour swatches in Figure 3. Often referred to as ‘fading curves’, these graphs may also depict changes in hue or darkening in some instances. When referenced to the original colour, the curve indicates a more rapid and pronounced colour change compared to the curve calculated from the residual colour after 10 Mlx·h of prior exposure. Results highlight the trend that colourants with previous exposure are generally less light-sensitive compared to their original, unexposed counterparts. For this specific scenario, the difference is quantified in the plot of the initial curves in Figure 5b. This graph shows the threshold of a JND as a horizontal dashed line. The intersection of the fading curves with this line reveals that 0.13 Mlx·h is required to induce a JND for the original material, whereas 1.9 Mlx·h is needed when starting from the residual (current) colour with 10 Mlx·h pre-exposure. In essence, the pre-exposed material demands an approximate 14-fold higher light dose to elicit a JND compared to the original.
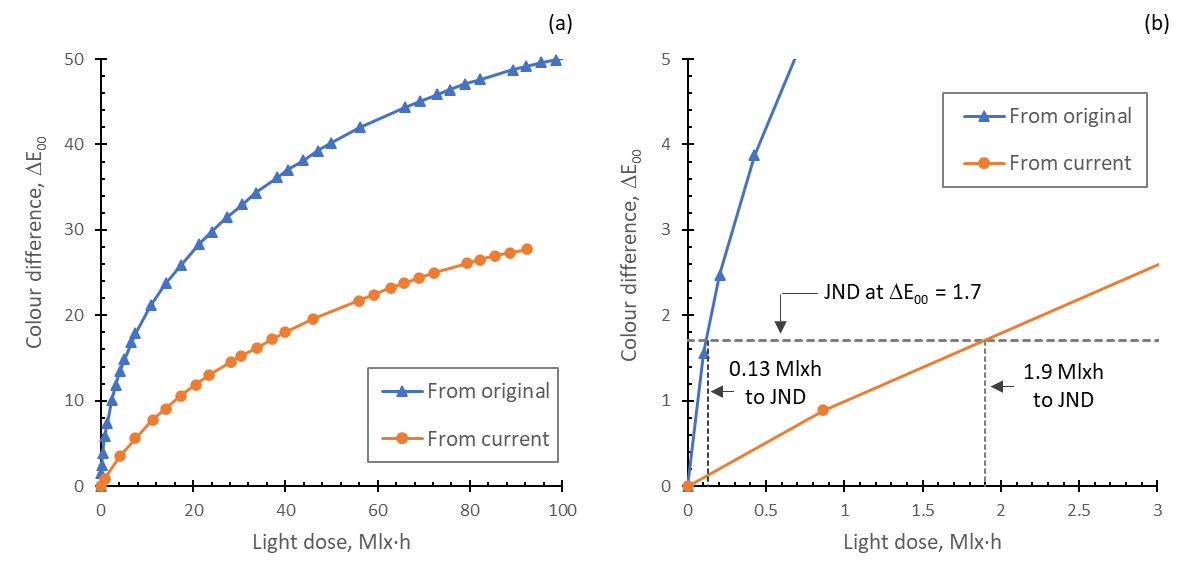
As a visual reference in HERIe, an approximate rendering of the ISO Grey Scale is presented as part of the calculator (Figure 6). Colour swatches are also given for the user-selected sample and ISO Blue Wools at defined exposure conditions. It is important to note that the rendering of Blue Wool swatches is currently based on colour measurements during exposure to the same warm-white LED test illuminant. The Blue Wool rate of colour change is different from the CIE 157 [7] responsivity to daylight with UV removed, despite both illuminants being ‘no UV’. This is due to the simple fact that light sources without UV do not have the same spectral power distributions in the visible range.
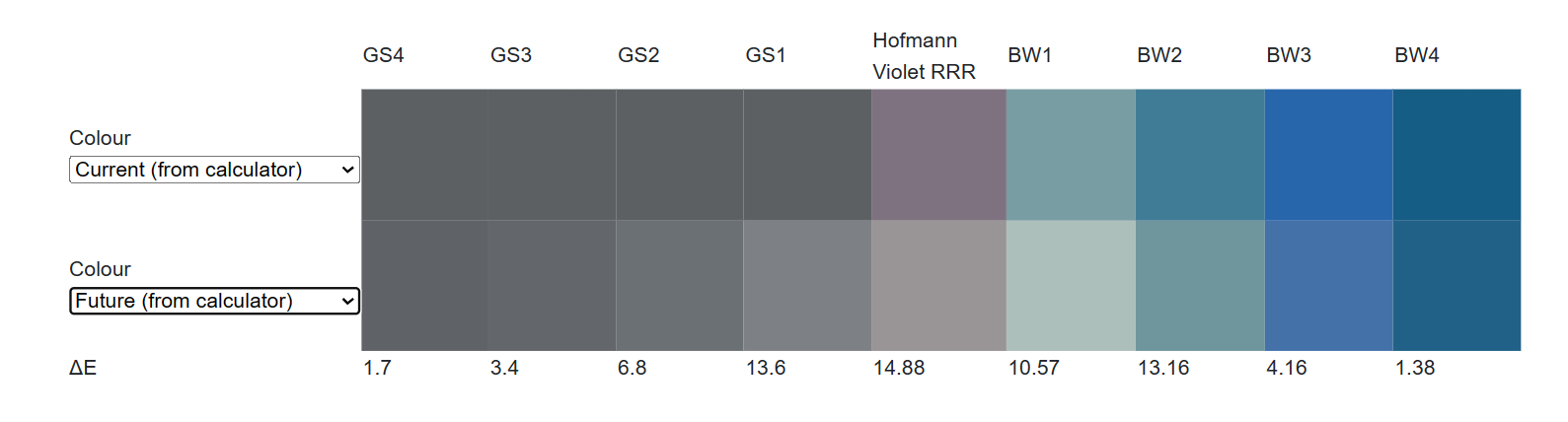
The final section of the calculator offers a comparison between samples in the collection, and a histogram of lightfastness, to move beyond a single-sample analysis. Figure 7 shows example histograms for the early synthetic dye collection using two approaches. The first (left) ranks the fastness of each sample using ISO Blue Wool responsivity values in CIE Technical Report 157 [7] (‘no UV’ condition) as a reference scale. An alternate approach is given in Figure 7 (right) where order-of-magnitude bins are used to construct the histogram of lightfastness, or dose causing a JND.
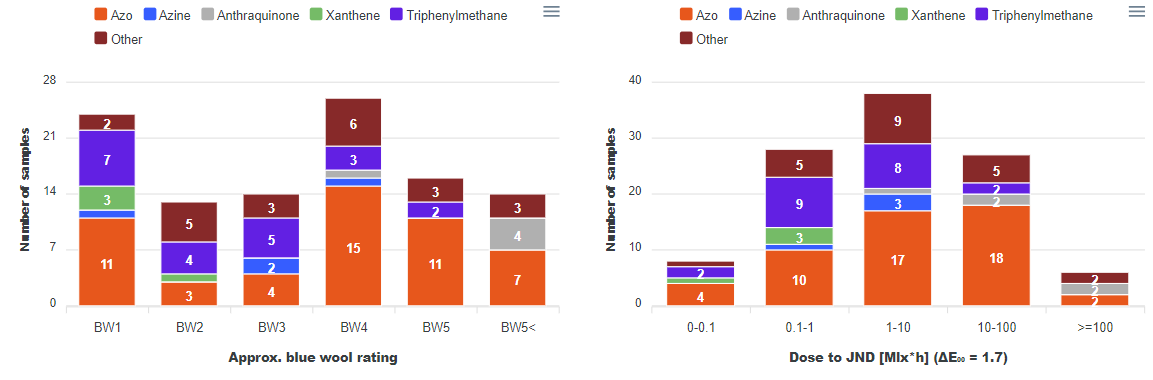
References
[1] Hagan E, Castro-Soto I, Breault M, Poulin J. The lightfastness of early synthetic organic dyes. Heritage Science. 2022;10:50. Available from: https://doi.org/10.1186/s40494-022-00675-9.
[2] Hagan E, Castro-Soto I, Breault M, Poulin J. Replication Data for: The lightfastness of early synthetic organic dyes [dataset]. 2022. Harvard Dataverse, V1. Available from: https://doi.org/10.7910/DVN/JQMYFM.
[3] Canadian Conservation Institute. Light Damage Calculator. Available from: https://app.pch.gc.ca/application/cdl-ldc/description-about.app?lang=en. Accessed Sept 26, 2023.
[4] Michalski S. The power of history in the analysis of collection risks from climate fluctuations and light. In Proceedings of the ICOM Committee for Conservation 17th Triennial Meeting, Melbourne, Australia 2014 Sep (pp. 15-19).
[5] American Association of Textile Chemists and Colorists. Gray Scale for Color Change. In: Technical manual of the American Association of Textile Chemists and Colorists. 1992;67:332-333.
[6] Hagan E, Poulin J. The effect of prior exposure on the lightfastness of early synthetic dyes on textiles. Heritage Science. 2022;10:138. Available from: https://doi.org/10.1186/s40494-022-00767-6.
[7] International Commission on Illumination. Control of damage to museum objects by optical radiation. CIE Technical Report 157:2004. Vienna: CIE; 2004.